- Department of Urology, Tianjin Medical University General Hospital, Tianjin, China
Background: There is still limited research on the association between immune cells and the risk of prostate cancer. Further investigations are warranted to comprehend the intricate associations at play.
Methods: We used a bidirectional two-sample Mendelian randomization (MR) analysis to investigate the causal relationship between immune cell phenotypes and prostate cancer. The summary data for immune cell phenotypes was derived from a study cohort, including 3,757 individuals from Sardinia with data on 731 immune cell phenotypes. The summary data for prostate cancer were obtained from the UK Biobank database. Sensitivity analyses were conducted, and the combination of MR-Egger and MR-Presso was used to assess horizontal pleiotropy. Cochran’s Q test was employed to evaluate heterogeneity, and the results were subjected to FDR correction.
Results: Our study identified two immune cell phenotypes significantly associated with the risk of prostate cancer, namely CD25 on naive-mature B cells (OR = 0.998, 95% CI, 0.997-0.999, P = 2.33E-05, FDR = 0.017) and HLA DR on CD14- CD16- cells (OR = 1.001, 95% CI, 1.000-1.002, P = 8.01E-05, FDR = 0.03). When adjusting FDR to 0.2, we additionally found six immune cell phenotypes influencing the incidence of prostate cancer. These include FSC-A on B cells (OR = 1.002, 95% CI, 1.001-1.002, P = 7.77E-04, FDR = 0.133), HLA DR on plasmacytoid dendritic cells (OR = 1.001, 95% CI, 1.000-1.001, P = 0.001, FDR = 0.133), CD14+ CD16- monocyte % monocytes (OR = 1.002, 95% CI, 1.001-1.003, P = 0.001, FDR = 0.133), and HVEM on effector memory CD4+ T cells (OR = 1.001, 95% CI, 1.000-1.002, P = 0.002, FDR = 0.169), which are positively correlated with the risk of prostate cancer. Conversely, CD25 on IgD+ B cells (OR = 0.998, 95% CI, 0.997-0.999, P = 0.002, FDR = 0.169) and Monocytic Myeloid-Derived Suppressor Cells AC (OR = 0.999, 95% CI, 0.999-1.000, P = 0.002, FDR = 0.17) are negatively correlated with the risk of prostate cancer.
Conclusion: This study has revealed causal relationships between immune cell phenotypes and prostate cancer, supplying novel insights that might aid in identifying potential therapeutic targets of prostate cancer.
Introduction
Prostate cancer is the second most common cancer in males, with an incidence rate second only to lung cancer. It is also the most common cancer in the male urinary system (1). The incidence of prostate cancer increases with the age of males (2). Additionally, there are significant differences in the incidence of prostate cancer based on race and geographic location. There is a 40-fold difference in incidence rates between African American males with the highest incidence and native Asian males with the lowest incidence in the United States (3). Western Europe, Northern Europe, North America, and other countries are high-incidence regions for prostate cancer, while regions such as Asia and North Africa have relatively lower incidence rates of prostate cancer (4). In addition to recognized age factors, risk factors for prostate cancer also include genetics, baldness, height, and others. Additionally, there are modifiable risk factors, including diet, smoking, and alcohol consumption (5).
An increasing number of studies have found a complex and close association between the immune system and cancer (6, 7). Many immunotherapies, such as immune checkpoint inhibitors or direct targeting of the tumor immune microenvironment, are used in cancer treatment, focusing on various immune cells within the immune system (8). Under normal circumstances, immune cells exert anti-tumor effects through immune surveillance and immune cytotoxicity. However, under certain conditions, certain immune cells may also promote the progression of tumors (9). This dual effect of immune cells occurs in various cancers. Studies have found a positive correlation between higher levels of FOXP3+ T cells mediating immune tolerance and lower levels of CD8+ T cells mediating cytotoxicity with the risk of breast cancer, colorectal cancer, and lung cancer in normal healthy populations (10). Similarly, research suggests that changes in the composition of immune cell tissues are linked to an elevated or reduced risk of specific cancers (11).. Immune cells also play a crucial role in prostate cancer (12–14). Studying the connection between immune cells and prostate cancer will contribute to exploring the mechanisms of prostate cancer, providing more potential treatment methods, and alleviating the burden on patients and society. Currently, there is still limited research on the association between immune cells and the risk of prostate cancer (15). More studies are needed to understand the complex connections involved.
Mendelian randomization (MR) is a method that utilizes genetic variations as instrumental variables (IVs) to assess observed causal relationships (16). The purpose of this approach is to simulate a randomized controlled trial, mitigating the influence of potential confounding factors in observational studies (17). Traditional observational studies determine disease risk factors by examining the relationship between exposure and outcomes. However, these studies may be limited in drawing valid causal conclusions due to confounding factors or reverse causation (18). Compared to traditional observational studies, MR is valuable for investigating causal relationships between risk factors and clinical diseases because genetic variations are randomly assigned at conception, typically unrelated to confounding factors, and unaffected by reverse causation (19). Our research aims to explore a causal relationship between immune cell traits and prostate cancer through a comprehensive two-sample bidirectional MR analysis.
Methods
Study design
The flowchart of the two-sample bidirectional Mendelian randomization (MR) analysis for immune cell phenotypes and the risk of prostate cancer is depicted in Figure 1. Initially, we employ immune cell phenotypes as the exposure to analyze which immune cell phenotypes may have potential causal relationships with the risk of prostate cancer. Subsequently, we use prostate cancer as the exposure and explore the potential reverse causal relationships with immune cell phenotypes. Single nucleotide polymorphisms (SNPs) are utilized as IVs in the study. The selected IVs satisfy three crucial assumptions: (1) IVs are associated with the risk exposure. (2) IVs are unrelated to any confounding factors influencing the exposure-outcome relationship. (3) IVs can only affect the outcome through the exposure and not through any other pathways (20). Any IVs violating the three major assumptions will be excluded.
Data sources
Immunology-related GWAS data sources
Our research data is derived from open GWAS databases and the UK Biobank database. The studies involved have all been approved by the local ethics committee. This study did not collect new data and does not require new ethical approval.
The summary statistics of immune cell phenotypes are derived from the GWAS database. GWAS data identifier from GCST90001391 to GCST90002121 (https://www.ebi.ac.uk/gwas/studies/GCST90002121). A cohort study involving 3,757 Sardinian individuals reported data on 22 million variants for 731 immune cell phenotypes. The 731 immune cell phenotypes consist of 118 absolute cell counts (AC), 192 relative counts (RC), 389 median fluorescence intensities (MFIs) of surface antigens, and 32 morphological parameters (MP).
This study involved the collection of peripheral blood from blood donors, which was then subjected to flow cytometry analysis following antibody staining. This process allowed for the identification and quantification of different cell subpopulations (21).
GWAS data sources for prostate cancer
Prostate cancer data were obtained from the UK Biobank database, comprising a study population of 462,933 individuals of European descent. The case group consisted of 3,269 individuals, and the control group included 459,664 individuals, involving 9,851,867 SNPs.
Selection of IVs
We set the threshold for SNPs related to immune cell phenotypes at P < 1 × 10-5. Additionally, we conducted a Linkage Disequilibrium (LD) check on these SNPs (r2 = 0.001 and kb = 10,000). The values of r2 or kb represent the degree of linkage disequilibrium between two loci, indicating that if there is LD between two loci, their allele frequencies are not independent but correlated in some way. For SNPs related to prostate cancer, we applied a threshold of P < 5 × 10-8 and similarly performed LD checks (r2 = 0.001 and kb = 10,000). We calculated the F-statistic for each SNP, and SNPs with low F-values (< 10) were removed as IVs to assess IV strength and mitigate weak instrument bias (22). Finally, we utilized the PhenoScanner database to exclude SNPs associated with potential confounding variables.
Statistical analysis
In order to explore the causal relationship between immune cell phenotypes and the risk of prostate cancer, this study primarily utilized the TwoSampleMR and MRPRESSO packages in R (4.2.3). The main conventional MR analysis methods employed included Inverse Variance Weighting (IVW), MR-Egger, Weighted Median, Weighted Mode, and MR-Presso. Depending on the specific situation, choose random or fixed-effect IVW. IVW, as the primary analytical method, aims to estimate the causal relationship between exposure factors and outcomes by combining the effects of various genetic variations, providing a comprehensive causal estimation. However, IVW has limitations as it relies on the three fundamental assumptions mentioned earlier and can only avoid the influence of confounding factors in the absence of horizontal pleiotropy. Therefore, when using the IVW method, the potential for horizontal pleiotropy must be considered (23).
In this study, we employed a combination of MR-Egger and MR-Presso to assess the presence of horizontal pleiotropy (P < 0.05 considered to indicate horizontal pleiotropy). Additionally, Cochran’s Q test was utilized to assess heterogeneity among the selected SNPs (P < 0.05 considered to indicate heterogeneity). Compared to MR-Egger, which detects and quantifies the degree of horizontal pleiotropy through intercept testing, MR-Presso can identify and address outliers beyond horizontal pleiotropy (24).
To explore reverse causality, the same methods were used for reverse MR analysis of immune cell phenotypes and prostate cancer. Moreover, considering the issue of multiple testing, FDR correction was performed using the online tool Bioladder. According to previous studies, FDR < 0.2 is considered suggestive of a causal relationship, while FDR < 0.05 is considered to indicate a significant causal relationship.
Results
The causal effect of immunophenotypes on prostate cancer
We first analyzed the causal effects of 731 immune cell phenotypes as exposure variables on prostate cancer, and the results of the analysis are shown in Figure 2. Our research findings revealed that two immune cell phenotypes are significantly associated with the risk of prostate cancer. Specifically, CD25 on naive-mature B cells (OR = 0.998, 95% CI, 0.997-0.999, P = 2.33E-05, FDR = 0.017) shows a significant negative correlation with the risk of prostate cancer, while HLA DR on CD14- CD16- cells (OR = 1.001, 95% CI, 1.000-1.002, P = 8.01E-05, FDR = 0.03) exhibits a significant positive correlation with the risk of prostate cancer. When adjusting the false discovery rate (FDR) to 0.2, we identified associations between six immune cell phenotypes and the risk of prostate cancer. Among them, FSC-A on B cells (OR = 1.002, 95% CI, 1.001-1.002, P = 7.77E-04, FDR = 0.133), HLA DR on plasmacytoid dendritic cells (OR = 1.001, 95% CI, 1.000-1.001, P = 0.001, FDR = 0.133), CD14+ CD16- monocyte % monocytes (OR = 1.002, 95% CI, 1.001-1.003, P = 0.001, FDR = 0.133), and HVEM on effector memory CD4+ T cells (OR = 1.001, 95% CI, 1.000-1.002, P = 0.002, FDR = 0.169) are positively correlated with the risk of prostate cancer, while CD25 on IgD+ B cells (OR = 0.998, 95% CI, 0.997-0.999, P = 0.002, FDR = 0.169) and Monocytic Myeloid-Derived Suppressor Cells AC (OR = 0.999, 95% CI, 0.999-1.000, P = 0.002, FDR = 0.17) are negatively correlated with the risk of prostate cancer. Subsequently, a horizontal pleiotropy test was conducted using MR-Egger and MR-Presso in combination. No horizontal pleiotropy was detected in the above results, and Cochran’s Q test revealed no heterogeneity in all outcomes. Following that, scatter plots and funnel plots also supported these findings (Supplementary Figures 1, 2). We further employed a heatmap for visual analysis of the research results. Initially, we filtered out the IDs of all positive results of immune cell phenotypes based on the p-values from the IVW method. Subsequently, different colors in Figure 3 represent the p-values of sensitivity analysis results for each immune cell phenotype.
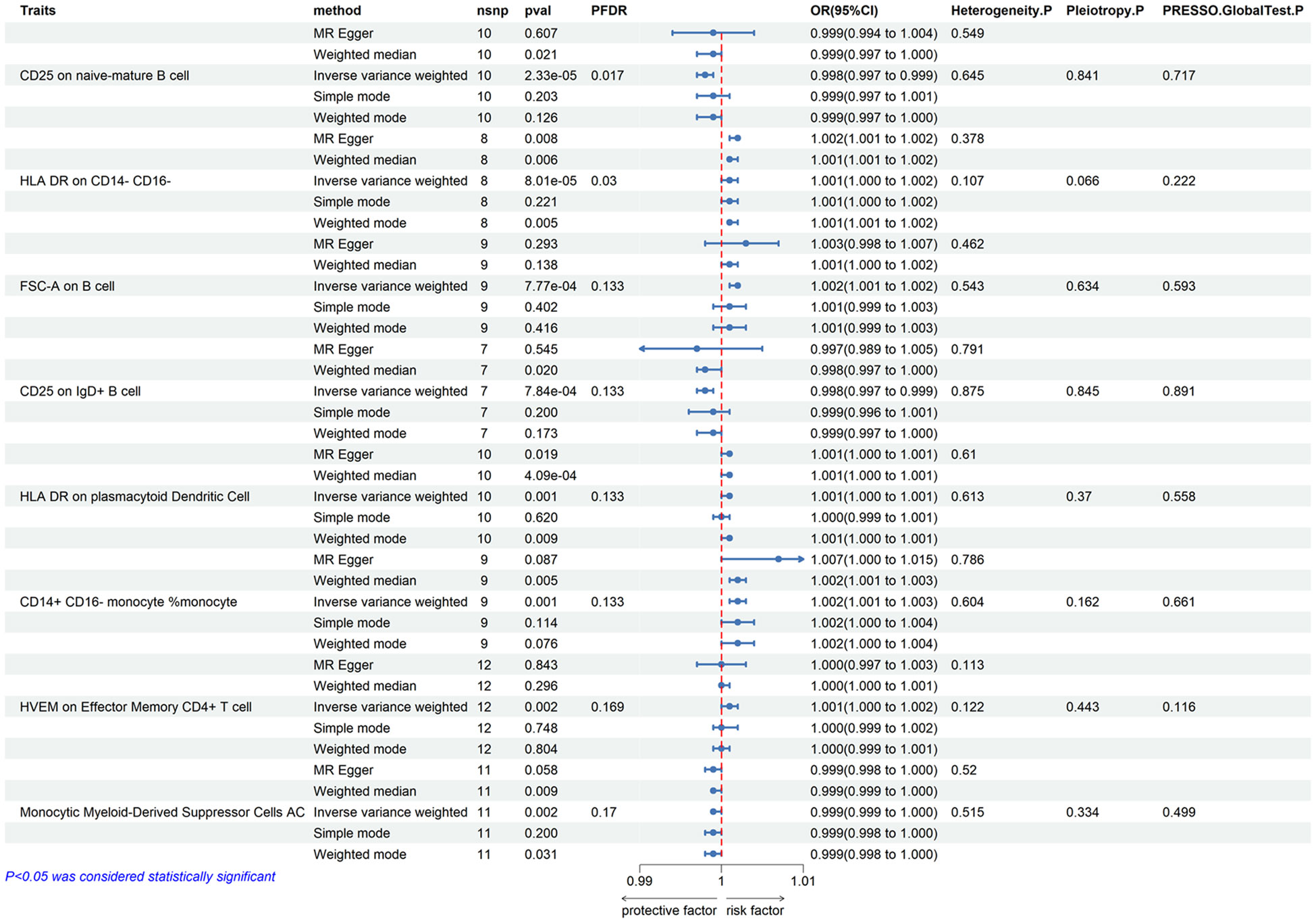
Figure 2 Forest plots showed the causal effect of immunophenotypes on prostate cancer. nsnp, nonsynonymous single-nucleotide polymorphism; OR, odds ratio; CI, confidence interval; PFDR, P value corrected by FDR.
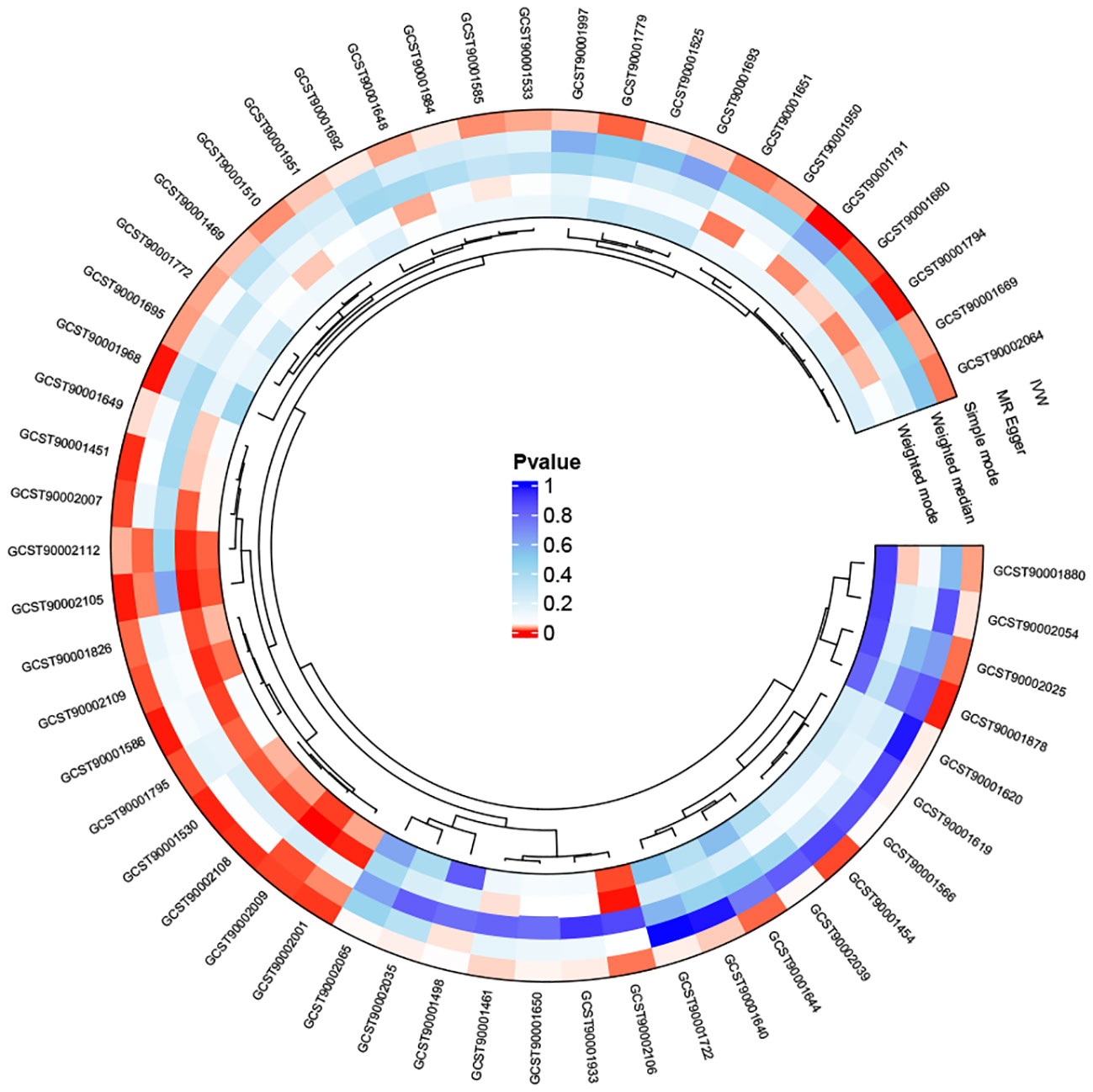
Figure 3 The heatmap depicting the IDs of immune cell phenotypes with positive results and the p-values from the sensitivity analysis: The outer circle represents the IDs of immune cell phenotypes, while the inner circle uses different colors to indicate the p-values of different sensitivity analysis results.
The causal effect of I prostate cancer on immunophenotypes
In the reverse MR analysis, we identified some positive results, but after FDR correction (FDR < 0.05), no statistically significant results were observed. Similarly, after adjusting to FDR < 0.2, no meaningful results were detected.
Discussion
Through a two-sample bidirectional MR study, we identified two immune cell phenotypes significantly associated with the risk of prostate cancer (FDR < 0.05). After adjusting for FDR < 0.20, an additional six immune cell phenotypes were found to be related to the risk of prostate cancer. In the reverse MR analysis, we also observed some positive results; however, after FDR correction, no significant correlations were identified.
Our study revealed a significant negative correlation between CD25 on naive-mature B cells and the risk of prostate cancer. Additionally, CD25 on IgD+ B cells also showed a negative correlation with the risk of prostate cancer, while FSC-A on B cells exhibited a positive correlation with the risk of prostate cancer. CD25 constitutes a component of the interleukin-2 (IL-2) receptor and is exhibited on the surface of diverse immune and non-immune cellular entities (25). The role of CD25 may vary significantly depending on its expression on different cell types. Regulatory T cells (Tregs) promote tumor progression, and CD25 is widely expressed on Tregs. Studies have found that depleting Tregs through anti-CD25 antibodies can exert an anti-tumor immune effect (26–28). However, recent research has found that agonists preserving the activity of CD25 can activate tumor-specific CD8 T cells, exerting an anti-tumor immune effect (29). These studies highlight the complex and crucial role of CD25 in tumor immunotherapy. Our study found that CD25 on naive-mature B cells and CD25 on IgD+ B cells are protective factors against prostate cancer. This finding aligns with similar conclusions from current research. Naive-mature B cells refer to B cells that have matured but have not been activated. In tumor immunity, naive-mature B cells may play an anti-tumor role by stimulating immune responses and assisting other immune cells (29). IgD+ B cells are a subset of B cells in the immune system, and they engage in immune responses through the surface expression of IgD. On B cells, IgD can coexist with other immunoglobulins, collectively regulating immune reactions (30). Studies have found the expansion of clonal B cells in both the blood and sentinel lymph nodes of prostate cancer patients, with a predominant presence of immature B cells in the blood (12). This reflects the protective role of B cells, including immature B cells, in prostate cancer.
Our study identified HLA DR on CD14- CD16-, CD14+ CD16- monocyte %monocyte, and HLA DR on plasmacytoid Dendritic Cell as risk factors for the incidence of prostate cancer. CD14 and CD16 are surface markers on immune cells, playing crucial roles in signal recognition, signal transduction, and enhancement of immune responses. They exert significant functions in both tumor and non-tumor diseases (31, 32). In the Monocyte panel, they were identified based on HLA-DR positivity. Pavlovic et al. has found reduced expression of the monocyte HLA-DR molecule in prostate cancer (33). A previous study indicated that enhancing monocyte function in the human body can be achieved by upregulating HLA-DR, contributing to anti-prostate cancer effects (34). Monocytes were categorized into classical cells (CD14+CD16−), non-classical cells (CD14−CD16+), and intermediate cells (CD14+CD16+) (21). Although the role of HLA-DR on CD14- CD16- is rarely mentioned, a recent study (35) still highlights its significant involvement in schizophrenia, warranting further attention. Currently, there is no dedicated study on the specific mechanism of HLA DR on CD14- CD16- in the development of prostate cancer. Further research is needed to validate our findings and explore the potential mechanisms involved. Plasmacytoid Dendritic Cells are multifunctional immune cells, and their clinical significance in the tumor microenvironment (TME) remains unclear (36), previous studies have found the immunosuppressive role of plasmacytoid dendritic cells in gastric cancer (37). The mechanisms by which they function in prostate cancer still require further exploration. Our study also found that Monocytic Myeloid-Derived Suppressor Cells (M-MDSCs) may be a potential protective factor in the development of prostate cancer. M-MDSC and polymorphonuclear MDSC (PMN-MDSC) are two main cellular subtypes of myeloid-derived suppressor cells (MDSCs). Morphologically, M-MDSCs resemble monocytes, while PMN-MDSCs have a multi-lobed nucleus similar to polymorphonuclear (PMN) cells. Moreover, these two subtypes express different surface molecules, and their distribution varies across different tumors (38). Previous studies have found that PMN-MDSCs contribute to the progression and immune evasion of prostate cancer (39, 40). Idorn et al. have identified increased expression of M-MDSCs in patients with castration-resistant prostate cancer, suggesting its involvement in the immune suppressive environment of prostate cancer patients (41). Further specific research is needed to elucidate these findings.
The strengths of our study include the first-time application of MR methods to investigate the relationship between immune cell phenotypes and prostate cancer. Our conclusions were derived under strict examination of horizontal pleiotropy, reducing the interference of confounding factors and the impact of reverse causality on the results. Additionally, our study identified immune cell phenotypes significantly associated with prostate cancer, which have been less explored in previous research. This may provide new insights for exploring potential immunotherapeutic targets in prostate cancer. Our study also has some limitations. Although we included 731 immune cell phenotypes in our research, there are still some immune cell phenotypes that could not be analyzed due to data limitations. Additionally, since the data sources are predominantly of European descent, limited to adults, and do not support stratification by gender and age, this may impact the generalizability and accuracy of the results. This study is based on a cohort study of individuals from Sardinia. The Sardinian population possesses unique genetic characteristics, and the study conclusions may not be applicable to broader populations. Future validation in larger and more diverse patient cohorts is necessary to ensure the robustness and generalizability of our conclusions. Furthermore, the selection of instrumental variables for immune cell phenotypes (P < 1×10−5) and the interpretation of reverse results (FDR < 0.2) are not as stringent. Finally, we hope that future research will involve larger sample sizes and more comprehensive Mendelian randomization studies to further explore the relationship between immune cell phenotypes and prostate cancer.
Conclusions
This study has revealed causal relationships between immune cell phenotypes and prostate cancer, supplying novel insights that might aid in comprehending the pathogenic mechanisms of prostate cancer and identifying potential therapeutic targets.
Data availability statement
The original contributions presented in the study are included in the article/Supplementary Material. Further inquiries can be directed to the corresponding author.
Ethics statement
Ethical approval was not required for the study involving humans in accordance with the local legislation and institutional requirements. Written informed consent to participate in this study was not required from the participants or the participants’ legal guardians/next of kin in accordance with the national legislation and the institutional requirements.
Author contributions
XH: Writing – review & editing, Methodology, Software, Writing – original draft. CR: Writing – original draft, Software. HZho: Writing – original draft, Investigation. ML: Data curation, Writing – review & editing. HZha: Data curation, Writing – review & editing. XL: Project administration, Supervision, Writing – review & editing.
Funding
The author(s) declare financial support was received for the research, authorship, and/or publication of this article. The study received funding from the Chinese National Natural Science Funds (82171594).
Acknowledgments
Thanks to GWAS database contributors for sharing the data.
Conflict of interest
The authors declare that the research was conducted in the absence of any commercial or financial relationships that could be construed as a potential conflict of interest.
Publisher’s note
All claims expressed in this article are solely those of the authors and do not necessarily represent those of their affiliated organizations, or those of the publisher, the editors and the reviewers. Any product that may be evaluated in this article, or claim that may be made by its manufacturer, is not guaranteed or endorsed by the publisher.
Supplementary material
The Supplementary Material for this article can be found online at: https://www.frontiersin.org/articles/10.3389/fendo.2024.1358416/full#supplementary-material
References
1. Rebello RJ, Oing C, Knudsen KE, Loeb S, Johnson DC, Reiter S, et al. Prostate cancer. Nat Rev Dis Primers (2021) 7:8. doi: 10.1038/s41572-021-00249-2
2. Belkahla S, Nahvi I, Biswas S, Nahvi I, Ben Amor N. Advances and development of prostate cancer, treatment, and strategies: A systemic review. Front Cell Dev Biol (2022) 10:991330. doi: 10.3389/fcell.2022.991330
3. Pernar CH, Ebot EM, Wilson KM, Mucci LA. The epidemiology of prostate cancer. Cold Spring Harbor Perspect Med (2018) 8:a030361. doi: 10.1101/cshperspect.a030361
4. Vickers AJ, Elfiky A, Freeman VL, Roach M 3rd. Race, biology, disparities, and prostate cancer. Eur Urol (2022) 81:463–5. doi: 10.1016/j.eururo.2022.02.007
5. Bergengren O, Pekala KR, Matsoukas K, Fainberg J, Mungovan SF, Bratt O, et al. 2022 Update on prostate cancer epidemiology and risk factors-A systematic review. Eur Urol (2023) 84:191–206. doi: 10.1016/j.eururo.2023.04.021
6. Gonzalez H, Hagerling C, Werb Z. Roles of the immune system in cancer: from tumor initiation to metastatic progression. Genes Dev (2018) 32:1267–84. doi: 10.1101/gad.314617.118
7. Hiam-Galvez KJ, Allen BM, Spitzer MH. Systemic immunity in cancer. Nat Rev Cancer (2021) 21:345–59. doi: 10.1038/s41568-021-00347-z
8. Tang T, Huang X, Zhang G, Hong Z, Bai X, Liang T. Advantages of targeting the tumor immune microenvironment over blocking immune checkpoint in cancer immunotherapy. Signal Transduction Targeted Ther (2021) 6:72. doi: 10.1038/s41392-020-00449-4
9. Peña-Romero AC, Orenes-Piñero E. Dual effect of immune cells within tumour microenvironment: pro- and anti-tumour effects and their triggers. Cancers (2022) 14:1681. doi: 10.3390/cancers14071681
10. Song M, Tworoger SS. Systemic immune response and cancer risk: filling the missing piece of immuno-oncology. Cancer Res (2020) 80:1801–3. doi: 10.1158/0008-5472.CAN-20-0730
11. Palomero L, Galván-Femenía I, de Cid R, Espín R, Barnes DR, Cimba, et al. Immune cell associations with cancer risk. iScience (2020) 23:101296. doi: 10.1016/j.isci.2020.101296
12. Saudi A, Banday V, Zirakzadeh AA, Selinger M, Forsberg J, Holmbom M, et al. Immune-activated B cells are dominant in prostate cancer. Cancers (2023) 15:920. doi: 10.3390/cancers15030920
13. Andersen LB, Nørgaard M, Rasmussen M, Fredsøe J, Borre M, Ulhøi BP, et al. Immune cell analyses of the tumor microenvironment in prostate cancer highlight infiltrating regulatory T cells and macrophages as adverse prognostic factors. J Pathol (2021) 255:155–65. doi: 10.1002/path.5757
14. Molina OE, LaRue H, Simonyan D, Hovington H, Têtu B, Fradet V, et al. High infiltration of CD209(+) dendritic cells and CD163(+) macrophages in the peritumor area of prostate cancer is predictive of late adverse outcomes. Front Immunol (2023) 14:1205266. doi: 10.3389/fimmu.2023.1205266
15. Kwon JTW, Bryant RJ, Parkes EE. The tumor microenvironment and immune responses in prostate cancer patients. Endocrine-related Cancer (2021) 28:T95–t107. doi: 10.1530/ERC-21-0149
16. Sekula P, Del Greco MF, Pattaro C, Köttgen A. Mendelian randomization as an approach to assess causality using observational data. J Am Soc Nephrol: JASN (2016) 27:3253–65. doi: 10.1681/ASN.2016010098
17. Bowden J, Holmes MV. Meta-analysis and Mendelian randomization: A review. Res Synthesis Methods (2019) 10:486–96. doi: 10.1002/jrsm.1346
18. Kim MS, Song M, Shin JI, Won HH. How to interpret studies using Mendelian randomisation. BMJ Evidence-Based Med (2023) 28:251–4. doi: 10.1136/bmjebm-2022-112149
19. Weith M, Beyer A. The next step in Mendelian randomization. eLife (2023) 12:e86416. doi: 10.7554/eLife.86416
20. Lor GCY, Risch HA, Fung WT, Au Yeung SL, Wong IOL, Zheng W, et al. Reporting and guidelines for mendelian randomization analysis: A systematic review of oncological studies. Cancer Epidemiol (2019) 62:101577. doi: 10.1016/j.canep.2019.101577
21. Orrù V, Steri M, Sidore C, Marongiu M, Serra V, Olla S, et al. Complex genetic signatures in immune cells underlie autoimmunity and inform therapy. Nat Genet (2020) 52:1036–45. doi: 10.1038/s41588-020-0684-4
22. Wang K, Han S. Effect of selection bias on two sample summary data based Mendelian randomization. Sci Rep (2021) 11:7585. doi: 10.1038/s41598-021-87219-6
23. Mbutiwi FIN, Dessy T, Sylvestre MP. Mendelian randomization: A review of methods for the prevention, assessment, and discussion of pleiotropy in studies using the fat mass and obesity-associated gene as an instrument for adiposity. Front Genet (2022) 13:803238. doi: 10.3389/fgene.2022.803238
24. Boehm FJ, Zhou X. Statistical methods for Mendelian randomization in genome-wide association studies: A review. Comput Struct Biotechnol J (2022) 20:2338–51. doi: 10.1016/j.csbj.2022.05.015
25. Peng Y, Tao Y, Zhang Y, Wang J, Yang J, Wang Y. CD25: A potential tumor therapeutic target. Int J Cancer (2023) 152:1290–303. doi: 10.1002/ijc.34281
26. Villanueva MT. Anti-CD25 antibody tips the T cell balance. Nat Rev Drug Discovery (2021) 20:18. doi: 10.1038/d41573-020-00206-w
27. Lee JH, Jung KH, Kim M, Lee KH. Cysteine-specific (89)Zr-labeled anti-CD25 IgG allows immuno-PET imaging of interleukin-2 receptor-α on T cell lymphomas. Front Immunol (2022) 13:1017132. doi: 10.3389/fimmu.2022.1017132
28. Zhang J, Fu Q, He Y, Lv H, Qian Y, Zhang Y, et al. Differences of circulating CD25(hi) bregs and their correlations with CD4 effector and regulatory T cells in autoantibody-positive T1D compared with age-matched healthy individuals. J Immunol Res (2022) 2022:2269237. doi: 10.1155/2022/2269237
29. Fuss P, Bal K, Jerzyńska J, Podlecka D, Stelmach W, Stelmach I. Association between environmental exposure and CD4+CD25+ regulatory T cells. Allergologia immunopathologia (2019) 47:43–6. doi: 10.1016/j.aller.2018.04.003
30. Rampoldi F, Donato E, Ullrich L, Deseke M, Janssen A, Demera A, et al. γδ T cells license immature B cells to produce a broad range of polyreactive antibodies. Cell Rep (2022) 39:110854. doi: 10.1016/j.celrep.2022.110854
31. Sakakura K, Takahashi H, Motegi SI, Yokobori-Kuwabara Y, Oyama T, Chikamatsu K. Immunological features of circulating monocyte subsets in patients with squamous cell carcinoma of the head and neck. Clin Immunol (Orlando Fla.) (2021) 225:108677. doi: 10.1016/j.clim.2021.108677
32. Prat M, Le Naour A, Coulson K, Lemée F, Leray H, Jacquemin G, et al. Circulating CD14(high) CD16(low) intermediate blood monocytes as a biomarker of ascites immune status and ovarian cancer progression. J Immunother Cancer (2020) 8:e000472. doi: 10.1136/jitc-2019-000472
33. Vuk-Pavlović S, Bulur PA, Lin Y, Qin R, Szumlanski CL, Zhao X, et al. Immunosuppressive CD14+HLA-DRlow/- monocytes in prostate cancer. Prostate (2010) 70:443–55. doi: 10.1002/pros.21078
34. Santiago KB, Rodrigues JCZ, de Oliveira Cardoso E, Conte FL, Tasca KI, Romagnoli GG, et al. Brazilian red propolis exerts a cytotoxic action against prostate cancer cells and upregulates human monocyte functions. Phytother Res PTR (2023) 37:399–409. doi: 10.1002/ptr.7618
35. Wang C, Zhu D, Zhang D, Zuo X, Yao L, Liu T, et al. Causal role of immune cells in schizophrenia: Mendelian randomization (MR) study. BMC Psychiatry (2023) 23:590. doi: 10.1186/s12888-023-05081-4
36. Adimora IJ, Wilson NR, Pemmaraju N. Blastic plasmacytoid dendritic cell neoplasm (BPDCN): A promising future in the era of targeted therapeutics. Cancer (2022) 128:3019–26. doi: 10.1002/cncr.34345
37. Tussiwand R. Plasmacytoid dendritic cells turn red! Nat Immunol (2023) 24:563–4. doi: 10.1038/s41590-023-01472-7
38. Özkan B, Lim H, Park SG. Immunomodulatory function of myeloid-derived suppressor cells during B cell-mediated immune responses. Int J Mol Sci (2018) 19:1468. doi: 10.3390/ijms19051468
39. Wen J, Huang G, Liu S, Wan J, Wang X, Zhu Y, et al. Polymorphonuclear MDSCs are enriched in the stroma and expanded in metastases of prostate cancer. J Pathol Clin Res (2020) 6:171–7. doi: 10.1002/cjp2.160
40. Li N, Liu Q, Han Y, Pei S, Cheng B, Xu J, et al. ARID1A loss induces polymorphonuclear myeloid-derived suppressor cell chemotaxis and promotes prostate cancer progression. Nat Commun (2022) 13:7281. doi: 10.1038/s41467-022-34871-9
41. Idorn M, Køllgaard T, Kongsted P, Sengeløv L, Thor Straten P. Correlation between frequencies of blood monocytic myeloid-derived suppressor cells, regulatory T cells and negative prognostic markers in patients with castration-resistant metastatic prostate cancer. Cancer Immunol Immunother CII (2014) 63:1177–87. doi: 10.1007/s00262-014-1591-2
Keywords: prostate cancer, immune cells, Mendelian randomization study, causality, reverse causality
Citation: Hao X, Ren C, Zhou H, Li M, Zhang H and Liu X (2024) Association between circulating immune cells and the risk of prostate cancer: a Mendelian randomization study. Front. Endocrinol. 15:1358416. doi: 10.3389/fendo.2024.1358416
Received: 19 December 2023; Accepted: 26 January 2024;
Published: 09 February 2024.
Edited by:
Anna Perri, Magna Græcia University of Catanzaro, ItalyReviewed by:
Xiaoqiang Wang, City of Hope, United StatesMarcos Edgar Herkenhoff, University of São Paulo, Brazil
Mudasir Rashid, Howard University Hospital, United States
Copyright © 2024 Hao, Ren, Zhou, Li, Zhang and Liu. This is an open-access article distributed under the terms of the Creative Commons Attribution License (CC BY). The use, distribution or reproduction in other forums is permitted, provided the original author(s) and the copyright owner(s) are credited and that the original publication in this journal is cited, in accordance with accepted academic practice. No use, distribution or reproduction is permitted which does not comply with these terms.
*Correspondence: Xiaoqiang Liu, xiaoqiangliu1@163.com
†These authors have contributed equally to this work